- The thermodynamic state of a system is defined
by specifying a set of measurable properties sufficient so that all remaining
properties are determined. Examples of properties: pressure, temperature,
density, internal energy, enthalpy, and entropy.
- For engineering purposes we usually want gross,
average, macroscopic properties (not what is happening to individual molecules
and atoms) thus we consider substances as continua -- the properties represent
averages over small volumes. For example, there are 1016 molecules
of air in 1 mm3 at standard temperature and pressure. (VW,
S & B: 2.2)
-
Intensive properties do not depend on mass (e.g.
p, T, r , v=1/r,
u and h); extensive properties depend on the total mass of the system
(e.g. V, M, U and H). Uppercase letters are usually used for extensive
properties. (VW, S & B: 2.3)
- Equilibrium: States of a system
are most conveniently described when the system is in equilibrium, i.
e. it is in steady-state. Often we will consider processes that change "slowly" -- termed quasi-equilibrium or quasi-static processes.
A process is quasi-equilibrium if the time rate of change of the process
is slow relative to the time it takes for the system to reach thermodynamic
equilibrium. It is necessary that a system be quasi-equilibrium before
applying many of the thermodynamics relations to that system.
If the system is not in equilibrium, then different parts of the system
exist at different states at the same time and it is not possible to define
one “state” of the system. Since many of the thermodynamic
relations relate to the state of the system, it is necessary that a state
can be defined for the system before applying them. (VW, S & B:
2.3-2.4)
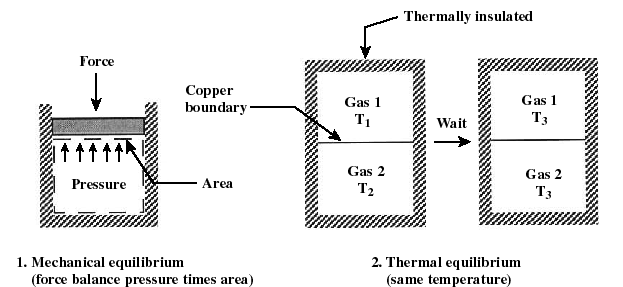
1. mechanical equilibrium 2.
thermal equilibrium
(force balances pressure times area) (same temperature)
Q2.3 (PDF)
Q2.4 (PDF)
- Two properties are needed
to define the state of any pure substance undergoing a steady or quasi-steady
process. (This is an experimental fact!) (VW, S & B: 3.1, 3.3)
-
For example for a thermally perfect gas (this is
a good engineering approximation for many situations, but not all (good
for p<<pcrit, and T>2Tcrit up to about
4pcrit). (VW, S & B: 3.4):
p
= RT
is volume per mol of gas, R
is the universal gas constant R
= 8.31kJ/Kmol-K.
Dividing by molecular weight,
p
/M
= (R
/ M )
T
where M
is the molecular weight of the gas.
Most often written as
pv = RT or p = rRT
where v is the specific volume and R
is the gas constant (which varies depending on the gas. R = 287J/kg - K
for air).
Thus, if we know p and T we know r,
if we know T and r,
we know p, etc.
Q2.5 (PDF)
- For thermodynamic processes
we are interested in how the state of a system changes. So typically we
plot the behavior as shown below. It is useful to know what a constant
temperature line (isotherm) looks like on a p-v diagram, what a constant
volume line (isochor) looks like on a T-p diagram, etc.

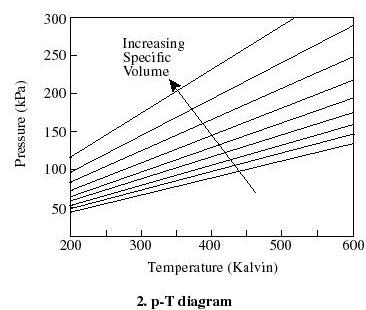
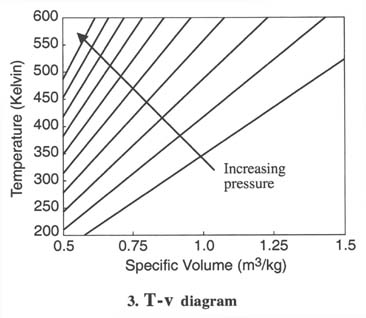
Q2.6 (PDF)
- Note that real substances may have phase changes
(water to water vapor, or water to ice, for example). Many thermodynamic
devices rely on these phase changes (liquid-vapor power cycles are used
in many power generation schemes, for example). You will learn more about
these in 16.050. In this course we will deal only with single-phase thermodynamic
systems.